Carbon dioxide levels in the Earth’s atmosphere stand today at 415 parts per million, which is significantly higher than concentrations have reached for at least the past eight hundred thousand years. Throughout this time, levels oscillated between 180 and 280 parts per million, until the mid-nineteenth century, when they began an inexorable rise. By the end of the century, if business as usual continues, carbon dioxide levels could be higher than at any time in the past fifty million years.1
Like many other concerned citizens, I have wondered what one person can possibly do to help stem the rise of carbon dioxide levels, warming temperatures, and accompanying species extinctions that characterize our Earth in the twenty-first century. Carbon is a two-part problem: we must simultaneously reduce combustion emissions and increase the removal of atmospheric carbon dioxide. As an individual, I can take action to reduce emissions (use more efficient LED bulbs, drive a more efficient car less often, use airplanes sparingly), but what about the other side of the equation? I have increasingly come to recognize that, as a landowner, the way I steward the vegetation on my property can make a difference to both sides of this problem.
I live in a small, residential neighborhood in an otherwise rural part of Connecticut. My property comprises a one-and-a-half-acre lot, about two-thirds wooded. The other third includes a yard (where the kids can kick a soccer ball), the house, and a gravel driveway that can accommodate several cars. Plants on my property, like those growing anywhere else, remove carbon dioxide from the atmosphere during photosynthesis and store it as carbon molecules in wood, roots, and leaves—a process known as carbon sequestration. Yet it’s surprising to learn just how much carbon dioxide is removed by the Earth’s natural vegetation: about 30 percent of all carbon emitted each year globally. With changes in the way we manage vegetation, this percentage could increase dramatically.2
Trees are key. An acre of temperate grassland and an acre of temperate forest store a similar amount of carbon in the soil, but a forest stores as much as seventeen to twenty times more carbon in the vegetation than does a grassland.3 Compare an acre of forest to an acre of lawn, and the carbon storage disparity is far greater. When we replace natural forest with fields, lawn, and other less-natural land covers (like roads, parking lots, and buildings), not only do we release huge amounts of carbon once stored in the trees into the atmosphere but we also sequester significantly less carbon going forward.
The Carbon in My Trees
I became curious about the role of my property in sequestering carbon and how much of a difference simple management decisions could make towards this end. How much carbon is stored in the trees on my property? To answer this question, I measured the diameter of every tree at least five inches in diameter at breast height and then used carbon estimation (“allometric”) equations devised by the United States Forest Service and researchers from Harvard Forest to estimate the total biomass in the trees.4 Plant tissue contains about 45 to 50 percent carbon, so dividing total biomass in half is a good approximation of the carbon storage in the plants.5 The results: 226 trees storing 84.3 tons of carbon total, including a forty-inch-diameter black oak (Quercus velutina) and a red oak (Quercus rubra) of nearly the same dimension. These big oaks comprise less than 1 percent of the trees on my lot but store a remarkable 13 percent of the carbon. The big oaks are not idle reservoirs of carbon either. A healthy red oak forty inches in diameter may add two-tenths of an inch to its trunk diameter each year—an imperceptible increase to even an observant naturalist—but a layer of carbon equal to adding an entire six-inch-diameter tree.6


The amount of carbon stored in the trees across my property is over 50 percent higher than in an average acre and a half of forest in Connecticut.7 The elevated levels can be attributed to the relatively high density of large trees in my woods, for which I have the past owners to thank. In addition to the two large oaks, seven other trees exceed twenty-seven inches in trunk diameter. A typical acre and a half of forest in Connecticut currently contains only one or two trees of this size.8 Ironically, the forest edge associated with residential properties appears to contribute to large tree growth. Trees within one hundred feet of a forest edge (which many of mine are) grow faster and thus are often larger—and store more carbon—than those in a forest interior because of reduced competition for light and greater leaf area.9 Hence, smaller residential properties can be surprisingly important contributors to carbon sequestration.
Natural Climate Solutions
As a property owner, I have many different options for how to manage the vegetation growing on my lot to increase the removal of carbon dioxide from the atmosphere and to reduce emissions. These practices are collectively referred to as natural climate solutions.10
By choosing not to convert the forest on my property into lawn or field (a practice known as avoided conversion), I refrain from emitting the carbon stored in those trees into the atmosphere as carbon dioxide: 310 tons of it. (Carbon dioxide emissions can be calculated by multiplying organic carbon—in this case, 84.3 tons—by 3.67). Three-hundred-ten tons of carbon dioxide is equivalent to the annual emissions of sixty-one cars.11 These are not insignificant numbers, and when multiplied across hundreds of thousands of small properties, the potential for avoided emissions is notable.
When retaining a forest, I have a range of management decisions that will affect the amount of carbon stored in my woods. At one extreme, I could remove all the adult trees and regenerate a young forest. At the other extreme, I could remove an occasional tree for firewood, a practice that falls within the category of reduced impact forest management, or, by practicing wildlands management, I could remove no trees at all. Not surprisingly, the latter scenarios result in a significantly greater amount of carbon storage in my woods than the former scenario. In fact, any tree removal on a property like mine reduces carbon storage below the potential maximum for that site (although it is also true that if I leave all my trees standing, which I mostly do, and obtain my firewood from another source, I transfer that carbon loss to another property). Hence, reduced impact forest management—retaining more trees, particularly large ones, for more time—can make an important difference in the amount of carbon that is retained in a forest.12
Decisions about tree retention in residential areas often involve mitigating risk to power lines. A few years ago, for instance, the power company asked for my permission to cut three healthy trees on the edge of my previous property: a red oak, white oak (Quercus alba), and pignut hickory (Carya glabra), all with trunk diameters of more than thirty inches. Removing three trees would not have resulted in any forest conversion on my property—indeed, there are young, small trees growing underneath these big ones—but the carbon stored on my property would have been reduced by about eight tons, equivalent to the annual emissions of almost six cars. A large tree thirty inches in diameter also removes about seventy times the quantity of pollutants (including carbon monoxide, ozone, nitrogen dioxide, and particulate matter) as a tree three inches in diameter.13 I decided that the trees were a relatively low risk to the powerlines and would provide more benefits if I allowed them to continue to grow and sequester carbon.
Wildlands management, the decision not to cut or mow any trees, has obvious limitations near houses, but it can be applied to more removed areas. In the relatively small number of wilderness areas and strict nature preserves in the northeastern United States, the trees store a disproportionately large amount of carbon relative to the region’s total forest area.14 Wildlands also have the potential to sequester much additional carbon. Because of a lengthy land-use history of forest clearance and intensive logging, northeastern forests are, on average, only about 20 to 30 percent of their maximum potential age (80 to 100 years versus 350 to 400 years) and store only about half their potential carbon. An eighty-year-old forest today can, in most cases—barring a major disturbance such as a windstorm or insect infestation—continue to accumulate carbon for at least the next two hundred years in live and dead trees and in the soil.15
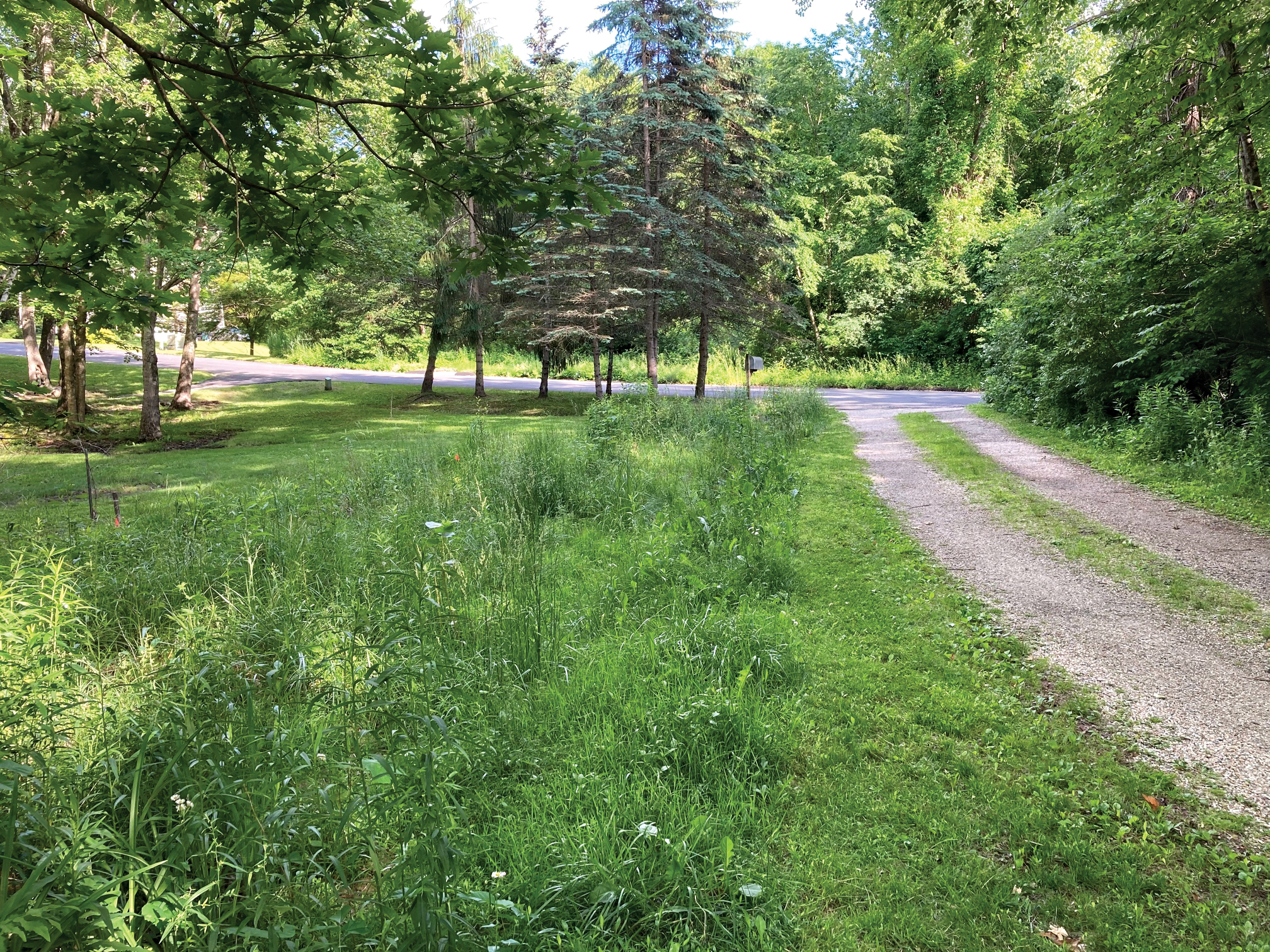
Another management option I have is reforestation: allowing an existing field to return to forest. I have begun reforestation on a small section of lawn along the edge of my property. Over the next fifteen years, this patch of regrowing forest may store as much as twenty-five times the aboveground carbon as the grassy lawn it replaced.16 Hence, reforestation has tremendous potential to sequester additional carbon on little-used pastures, agricultural fields, vacant lots, municipal fields, and small lawns on residential properties.17 There is a good reason for this potential: a site in which the trees have been removed—either recently or long ago—is in a deep carbon debt because the land stores a fraction of the carbon it once stored as a forest.
Energy Use
Trees, of course, also have other climate-related implications for my property. Trees standing within sixty feet of my house reduce home energy expenditure and carbon emissions by cooling the house in summer and insulating it from cold winds in winter. Not surprisingly, large trees provide significantly greater energy reductions than do small trees. A thirty-inch-diameter red maple located on the west side of a house would reduce carbon dioxide emissions by almost seven-fold compared to a two-inch-diameter red maple that is similarly placed.18
One caveat is that trees, especially conifers, located on the south side of a house increase winter fuel use by blocking solar radiation; but the drawbacks are generally offset by the substantial year-round benefits of trees located on the other three sides of a house. For example, if a thirty-inch white pine was growing on the south side of my house, it would increase winter fuel use slightly, while still providing some summer cooling, resulting in an estimated 10 pounds of additional carbon dioxide emitted annually. But the same tree on the north side of the house would reduce winter fuel use—and provide greater summer cooling—resulting in the reduced emissions of an estimated 335 pounds of carbon dioxide annually.19 Trees, therefore, play an important role not only in sequestering and storing carbon but also in reducing household carbon emissions.
Habitat and Biodiversity
Natural climate solutions can also provide important forest habitat. Trees, as they age and grow larger, provide nesting and denning sites for a host of birds and mammals.20 They create deadwood that provides food for insects and develop large crowns that supply an abundant seed source. Even scattered trees with trunks at least sixteen-to-twenty inches in diameter in an urban setting can have outsized effects on bird diversity and abundance—a role that has caused researchers to describe large urban trees as “biodiversity hotspots.”21
Reforestation of fields and lawns can provide additional young forest habitat (when the trees are fifteen years of age or younger), an ephemeral and uncommon habitat in the northeastern United States. Several species of birds (like chestnut-sided warbler, prairie warbler, indigo bunting, and brown thrasher) and the rare New England cottontail prefer dense, low woody vegetation found in young forests, shrublands, and disturbed open woods and are generally not found in closed forests.22
Depending on how many trees are retained or regrown on a property, and where the property is located, a small parcel may serve as a green oasis in an otherwise developed environment, or as an uncommon vegetation structure in a landscape of mostly mature forest or field, or as an extension of a larger forested patch. My property best exemplifies the last scenario, as it abuts one hundred acres of contiguous forest. I frequently see and hear wood thrushes, veeries, barred owls, and pileated woodpeckers on my property. These species generally prefer mature forests or are associated with larger trees, and the wood thrush is listed as globally “near threatened” by the International Union of Conservation of Nature.23 Such species would almost certainly avoid my property if I converted my woods into lawn. Given that North America has lost almost 30 percent of its total bird population in the past fifty years, the natural climate solutions presented here applied across a multitude of small properties could make a real difference in stemming these population declines.24
Management for Natural Climate Solutions
In general, the less I manage my property, the more climate benefits it will provide. Some tending, however, is important to allow trees to continue growing to their full potential. Lianas like the non-native oriental bittersweet (Celastrus orbiculatus), which thrive in the edge habitats characteristic of residential properties, are best cut and removed when they are growing up trees and over shrubs. Bittersweet will reduce the growth rate (and carbon uptake) and eventually kill trees by intercepting much of the sunlight in the canopy and by strangling the trunk.25 The native poison ivy (Toxicodendron radicans) and grape (Vitis spp.) are generally more benign than bittersweet, but they function similarly and can proliferate in edge habitats, so I generally cut these vines at the base of my trees to give the trees every advantage to remain healthy and sequester the most carbon.
With less management, tree branches inevitably grow close to my house and into my driveway and need to be trimmed periodically. After trimming, I deposit the branches in a brush pile or scatter them into the woods rather than chipping them or carting them away. Brush piles serve as cover and den habitat for a variety of small animal species such as red-backed salamanders, red-spotted newts, wood frogs, wrens, white-throated sparrows, juncos, and box turtles.26
Trees will also die over time from insects, pathogens, and other causes and can be a hazard if houses, cars, or recreational spaces are in the fall zone. Common sense dictates that these should be cut down. But if dead trees are not a hazard, they provide considerable benefits if left standing and are not an indication that the forest is “unhealthy” and needs to be fixed. Though no longer sequestering additional carbon, standing dead trees continue to store existing carbon, often for decades, as the carbon is released slowly via decomposition.27 Dead trees also provide habitat for cavity-nesting birds and mammals and serve as an abundant source of insect food for woodpeckers and other bark-gleaning birds like nuthatches. On my property, a standing dead elm tree (Ulmus americana) is used each year by a pair of yellow-bellied sapsuckers as a nest site.

When I need to remove a dead tree that poses a hazard, I move it into the woods after cutting it. Similarly, when large branches and trees fall during storms, I move them off the driveway and lawn and into the woods and use some for firewood. I also resist cleaning up downed branches and trees in the woods. Downed logs serve as habitat for a host of animals, replenish nutrients and carbon to the soil, act as germination sites for new tree seedlings, and store large amounts of carbon, often for decades.28
Reforestation also requires little to no management. Tree growth is the default process in the Northeast, and the vegetation will naturally self-organize into a forest over time if a landowner simply stops mowing a lawn or field. The cessation of mowing will also add to the carbon benefits of reforestation by eliminating a significant source of emissions.29 A tall grass layer will inhibit tree growth because of competition and shading, and therefore shrubs, even thorny invasives like multiflora rose (Rosa multiflora), will generally facilitate tree seedling growth by reducing the grass layer and protecting the seedlings from deer browsing.30 In most cases, tree seedlings will eventually grow above the shrubs and reduce shade-intolerant shrub species; however, in some instances, a dense shrub layer can suppress further tree growth beneath it.31 In such cases, selectively removing some shrubs can be beneficial. Planting trees can supplement and speed up natural reforestation, but it can be expensive and labor-intensive, and is ultimately unnecessary unless a homeowner is interested in an immediate screen planting or a particular species that does not grow nearby.
The Final Look
Ultimately, implementing natural climate solutions is an exercise in restraint and may challenge a homeowner’s sense of aesthetics. Indeed, given the choice, many homeowners prefer a relatively open, tidy property, with a few trees, long views, and unobstructed sunsets. But a property stewarded for natural climate solutions can offer a beauty not found in more open landscapes. On my property, I appreciate the delicate beams of light that pass through the foliage and columnar tree trunks in the early or later parts of a summer day; the brilliant reds, yellows, and oranges that envelop the property each autumn; and multitudes of snow- or ice-covered branches on a winter day. For six months of the year, when the leaves have fallen from the deciduous trees, the views lengthen and sunsets emerge. Even during the growing season, I enjoy surprisingly long views because most of the foliage on the large deciduous trees is above rather than below the sightlines.
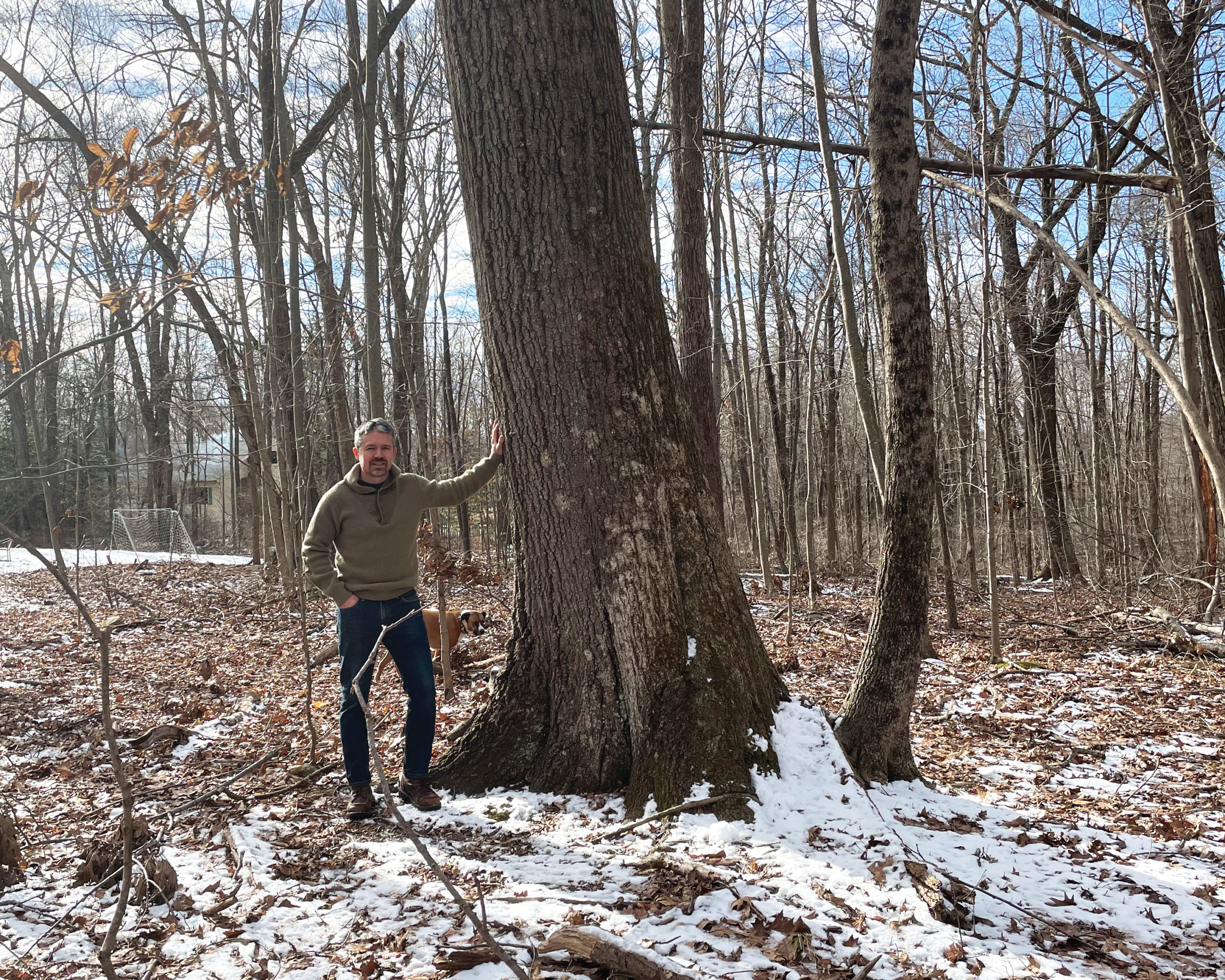
In the small area where I have begun reforestation, sightlines are reduced and the brushy patch of tall grass, young trees, and shrubs look unkempt compared to my neighbors’ adjacent, close-cropped lawn. Yet this management decision comes with other aesthetic rewards: insects busily foraging on the tall goldenrods that bloom in late summer and the flash of goldfinches and white-throated sparrows drawn to the seed source in this brushy new habitat.
In the end, there is a natural beauty that accompanies the climate and biodiversity benefits of leaving more vegetation intact. Faced with runaway carbon dioxide levels and a rapidly warming climate, property owners can leverage the carbon-absorbing power of trees by keeping them standing and growing and by allowing an existing field to revert to forest by not mowing. In this way, we can play an important role in the solution by doing less and letting nature do more.
Endnotes
1 Current carbon dioxide levels are posted on https://www.co2.earth/. The long history of carbon dioxide levels on earth are discussed in Lindsey, R. 2020. Climate change: Atmospheric carbon dioxide. NOAA Climate.gov. Retrieved from https://www.climate.gov/news-features/understanding-climate/climate-change-atmospheric-carbon-dioxide; and in Kolbert, E. 2014. The sixth extinction: An unnatural history. New York: Henry Holt and Company.
2 Harris, N. L. 2020. Young forests capture carbon quicker than previously thought. World Resources Institute. Retrieved from https://www.wri.org/blog/2020/09/carbon-sequestration-natural-forest-regrowth
3 Adams, J. M., Faure, H. F. D. L., Faure-Denard, L., McGlade, J. M., and Woodward, F. I. 1990. Increases in terrestrial carbon storage from the Last Glacial Maximum to the present. Nature, 348(6303): 711–714.
4 Harvard Forest. 2013. Schoolyard LTER database: Tree biomass equations. Retrieved from https://harvardforest.fas.harvard.edu/sites/harvardforest.fas.harvard.edu/
files/Tree%20Biomass%20Equations%202013.pdf
5 Schlesinger, W. H. 1997. Biogeochemistry: An analysis of global change. San Diego: Academic Press.
6 Stephenson, N. L., Das, A. J., Condit, R., Russo, S. E., Baker, P. J., Beckman, N. G., … and Zavala, M. A. 2014. Rate of tree carbon accumulation increases continuously with tree size. Nature, 507(7490): 90–93.
7 Data on average carbon storage in Connecticut forests was calculated using the USDA Forest Service, Forest Inventory and Analysis Program EVALIDATOR tool. Retrieved from http://apps.fs.usda.gov/Evalidator/evalidator.jsp
8 Data on large tree density in Connecticut forests was calculated using the USDA Forest Service, Forest Inventory and Analysis Program EVALIDATOR tool.
9 Reinmann, A. B., Smith, I. A., Thompson, J. R., and Hutyra, L. R. 2020. Urbanization and fragmentation mediate temperate forest carbon cycle response to climate. Environmental Research Letters, 15(11): 114036.
10 Fargione, J. E., Bassett, S., Boucher, T., Bridgham, S. D., Conant, R. T., Cook-Patton, S. C., … and Gu, H. 2018. Natural climate solutions for the United States. Science Advances, 4(11): eaat1869; Foster, D., Aber, J., Cogbill, C., Hart, C., Colburn, E., D’Amato, A., … and Thompson, J. 2010. Wildlands and woodlands: A vision for the New England landscape. Cambridge: Harvard University Press; Moomaw, W. R., Masino, S. A., and Faison, E. K. 2019. Intact forests in the United States: Proforestation mitigates climate change and serves the greatest good. Frontiers in Forests and Global Change, 2: 27.
11 The emission of the entire 84.3 tons of carbon (310 tons of carbon dioxide) into the atmosphere assumes that all cut trees would be chipped and burned and none would be used for wood products. If the trees were used for wood products, approximately 20–25 percent of the tree carbon would be stored in wood. See: Nunery, J. S., and Keeton, W. S. 2010. Forest carbon storage in the northeastern United States: Net effects of harvesting frequency, post-harvest retention, and wood products. Forest Ecology and Management, 259(8): 1363–1375; United States Environmental Protection Agency. 2019. Greenhouse gases equivalencies calculator – Calculations and references. Retrieved from https://www.epa.gov/energy/greenhouse-gases-equivalencies-calculator-calculations-and-references
12 Three key sources were used for this paragraph: Catanzaro, P., and D’Amato, A. 2019. Forest carbon: An essential natural solution for climate change. Amherst: University of Massachusetts; Fargione, et al. Natural climate solutions for the United States; Nunery and Keeton. Forest carbon storage in the northeastern United States.
13 Nowak, D. J. 2000, April. Tree species selection, design, and management to improve air quality. In 2000 ASLA annual meeting proceedings (pp. 23–27). Washington, DC: American Society of Landscape Architects.
14 Lu, X., Kicklighter, D. W., Melillo, J. M., Yang, P., Rosenzweig, B., Vörösmarty, C. J., … and Stewart, R. J. 2013. A contemporary carbon balance for the northeast region of the United States. Environmental science and technology, 47(23): 13230–13238.
15 Information on age and carbon storage of old growth forests in the northeastern United States was drawn from: Keeton, W. S., Whitman, A. A., McGee, G. C., and Goodale, C. L. 2011. Late-successional biomass development in northern hardwood-conifer forests of the northeastern United States. Forest Science, 57(6): 489–505; McGarvey, J. C., Thompson, J. R., Epstein, H. E., and Shugart Jr., H. H. 2015. Carbon storage in old-growth forests of the Mid-Atlantic: Toward better understanding the eastern forest carbon sink. Ecology, 96(2): 311–317. The average age of the region’s forests was calculated using the USDA Forest Service, Forest Inventory and Analysis Program EVALIDATOR tool. Retrieved from http://apps.fs.usda.gov/Evalidator/evalidator.jsp
16 See appendix B in: Smith, J. E., Heath, L. S., Skog, K. E., and Birdsey, R. A. 2006. Methods for calculating forest ecosystem and harvested carbon with standard estimates for forest types of the United States. General Technical Report NE-343. Newtown Square, PA: US Department of Agriculture, Forest Service, Northeastern Research Station.
17 Cook-Patton, S. C., Leavitt, S. M., Gibbs, D., Harris, N. L., Lister, K., Anderson-Teixeira, K. J., … and Griscom, H. P. 2020. Mapping carbon accumulation potential from global natural forest regrowth. Nature, 585(7826): 545–550.
18 Energy savings from trees near houses was estimated using i-Tree tools. Retrieved from https://www.itreetools.org/tools
19 Estimated using i-Tree tools.
20 Ranius, T., Niklasson, M., and Berg, N. 2009. Development of tree hollows in pedunculate oak (Quercus robur). Forest Ecology and Management, 257(1): 303–310.
21 Stagoll, K., Lindenmayer, D. B., Knight, E., Fischer, J., and Manning, A. D. 2012. Large trees are keystone structures in urban parks. Conservation Letters, 5(2): 115–122.
23 BirdLife International. 2017. Hylocichla mustelina (amended version of 2016 assessment). The IUCN Red List of Threatened Species 2017: e.T22708670A111170926. https://dx.doi.org/10.2305/IUCN.UK.2017-1.RLTS.T22708670A111170926.en. Downloaded on 13 January 2020.
24 Rosenberg, K. V., Dokter, A. M., Blancher, P. J., Sauer, J. R., Smith, A. C., Smith, P. A., … and Marra, P. P. 2019. Decline of the North American avifauna. Science, 366(6461): 120–124; DeGraaf, R. M., Yamasaki, M., Leak, W. B., and Lester, A. M. 2006. Technical guide to forest wildlife habitat management in New England. Lebanon, NH: University Press of New England.
25 Webster, C. R., Jenkins, M. A., and Jose, S. (2006). Woody invaders and the challenges they pose to forest ecosystems in the eastern United States. Journal of Forestry, 104(7): 366–374.
26 DeGraaf, et al. Technical guide to forest wildlife habitat management in New England.
27 Krebs, J., Pontius, J., and Schaberg, P. G. 2017. Modeling the impacts of hemlock woolly adelgid infestation and presalvage harvesting on carbon stocks in northern hemlock forests. Canadian Journal of Forest Research, 47(6): 727–734.
28 McGarvey, et al. Carbon storage in old-growth forests of the Mid-Atlantic; DeGraaf, et al. Technical guide to forest wildlife habitat management in New England.
29 Jo, H. K., and McPherson, G. E. 1995. Carbon storage and flux in urban residential greenspace. Journal of Environmental Management, 45(2): 109–133.
30 Holl, K. D. 2002. Effect of shrubs on tree seedling establishment in an abandoned tropical pasture. Journal of Ecology, 90: 179–187; Meiners, S. J., and Martinkovic, M. J. 2002. Survival of and herbivore damage to a cohort of Quercus rubra planted across a forest—old-field edge. The American Midland Naturalist, 147(2): 247–255.
31 Banasiak, S. E., and Meiners, S. J. 2009. Long term dynamics of Rosa multiflora in a successional system. Biological Invasions, 11(2): 215–224; Niering, W. A., Dreyer, G. D., Egler, F. E., and Anderson Jr, J. P. 1986. Stability of Viburnum lentago shrub community after 30 Years. Bulletin of the Torrey Botanical Club, 113(1): 23–27.
Edward K. Faison is senior ecologist at Highstead in Redding, Connecticut. His work focuses on deer and moose interactions with forests, long-term forest change, and natural climate solutions.
Citation: Faison, E. K. 2021. Backyard Climate Solutions. Arnoldia, 78(3): 28–37.